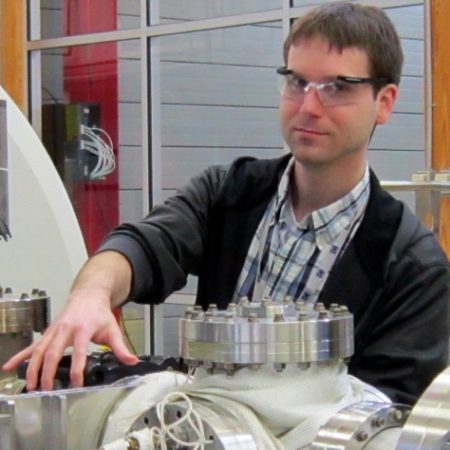
Florida State University scientists are offering a new understanding of how an intriguing nanomaterial — metallofullerene — is formed in a recently published research study.
Metallofullerenes are part of the carbon family, and kin to what’s popularly known as buckyballs. Buckyballs, or fullerenes, are hollow, soccer-ball-shaped, spherical cages that represent a basic form of carbon. The empty spaces in the fullerenes can trap metal atoms, resulting in metallofullerenes.
“Metallofullerenes are a unique form of molecular nanocarbon,” says FSU chemist Paul Dunk, a co-author of the study. “They are potentially useful in a number of biomedical diagnostics, in particular as MRI contrast agents.”
The published findings could help pave the way for metallofullerene-based applications that range from biomedicine to renewable energy. The article, “Bottom-up formation of endohedral metallofullerenes is directed by charge transfer,” was published in the December issue of Nature Communications.
“Under certain conditions, metallofullerenes can have spectacular properties that make them prized as advanced materials for an array of technologies, such as conversion of sunlight into electricity and as possible components of molecular electronics,” Dunk said.
The metal-encapsulated carbon cages may even be important cosmic molecules, forming in stellar environments and stardust.
To discover how metallofullerenes are synthesized in a lab, the research team relied on the high magnetic field instrumentation available at the Ion Cyclotron Resonance facility at the National High Magnetic Field Laboratory. The international team included: Florida State’s Harry Kroto, recipient of the 1996 Nobel prize in chemistry for the discovery of fullerenes; MagLab chemists; and scientists from the University Rovira i Virgili in Spain and Nagoya University in Japan.
Metallofullerenes are made by an astonishingly simple process: Mix graphite and a metal, and then vaporize it into soot, which looks like the black stuff from a candle flame. From that soot, metallofullerenes are mysteriously found.
“By vaporizing carbon and metal under the right conditions, these fascinating materials spontaneously assemble,” Dunk said. “But if the primary way in which they form is not even known, it’s difficult to figure out how to better produce these exciting molecules.”
While empty cages such as Buckminsterfullerene, C60, are available in ton quantity today, metallofullerenes suffer from limited quantities, thereby hindering research that fully explores the material.
“We first saw evidence for metallofullerenes just days after the discovery of Buckminsterfullerene in 1985, but we were not sure how they even formed. It was just amazing that they even did,” Kroto said. “That was nearly three decades ago. Despite major advances over the past 10 years, the formation process has proved very challenging because it occurs in a blink of an eye.”
To uncover the long-standing puzzle, researchers used a laser to blast graphite doped with metal, and the complex products formed were analyzed by the lab’s 9.4-tesla Fourier transform ion cyclotron resonance mass spectrometer. The powerful analysis technique allowed the team to meticulously study metallofullerene formation with a whopping 90 different elements, nearly all available elements of the periodic table.
The unprecedented results allowed the formation mechanism to be pieced together, building on recent pioneering work on empty cages from the same group.
Previously, it was predicted that flat sheets of carbon should be ejected from graphite and close up to form giant metallofullerenes, which could then hypothetically “shrink” into medium-sized cages that are most commonly used in biomedicine and technology.
However, the researchers observed an opposite outcome in their experiments. They found a metal atom initially nucleates carbon to form very small metallofullerenes, which then grow into the well-known larger cage sizes.
The type of metal encapsulated appeared to significantly affect how fast the small metallofullerenes grew into the most useful medium-sized cages, which could help explain the low yield of metallofullerenes by use of typical synthesis methods.
Clarification of the how molecular construction of these metal-encapsulated carbon cages occurs should help to open up new directions in nanotechonolgy.
“We hope these results will be useful in devising new production strategies to fully realize metallofullerene applications and further explore their amazing properties, which would certainly benefit society,” Dunk said.