Nanoparticles show enormous potential for therapeutics due to unique properties that emerge in nanostructured materials. Quantum dots, for instance, show robust size-dependent fluorescence that allows potential applications in medical imaging. Silicon is a particularly promising material for medical applications of quantum dots because bulk silicon is non-toxic and fluorescence of silicon nanoparticles can be tuned to emit at infrared frequencies where human tissue is transparent.
The question as to whether nanomaterials are toxic has plagued nanoparticle applications in medicine since certain gold and silica nanoparticles were found to be cytotoxic in the 1990s. Because there are so many different nanomaterials that could be made from the same material, asking if nanoparticles are toxic is like asking if chemicals toxic. Some are, some aren’t, and they need to be tested to find out.
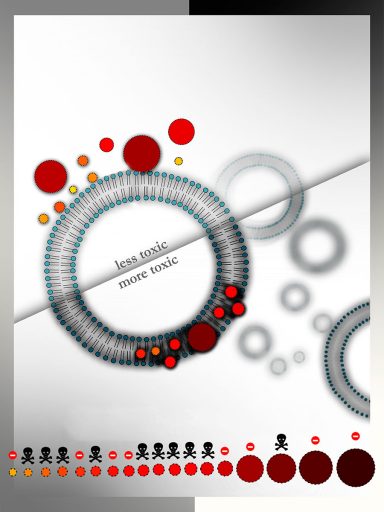
Unlike organic compounds, however, nanoparticles are usually synthesized as complex mixtures of different sizes and shapes, which can’t currently be described as one chemical structure. To further complicate the problem, nanoparticles are often synthesized in a water insoluble form.
For this reason, most nanochemists have shifted to coating nanoparticles with water soluble ligands for biological applications. However, lipophilic nanoparticles are commonly used in topical consumer products such as cosmetics and sunscreen, but only after being thoroughly tested for safety.
In order to investigate the biological activity of silicon nanoparticles, scientists at Florida State University, the University of Toronto and Karlsruhe Institute of Technology collaborated to measure the cytotoxicity of lipophilic silicon nanoparticles that had been size-separated by chemical purification methods and encapsulated into liposomes for delivery to HeLa cells, a standard cancer cell model.
Previous studies of this sort had relied on varying synthetic procedures, but this approach allows a more rigorous purification of the bioactive nanomaterials. The particle sizes ranged from 1.5 nanometers to 5 nanometers in diameter.
Surprisingly, we found that 10 out of 18 fractions of the purified nanomaterials were significantly more toxic than the crude mixture or the other fractions. The measurements were repeated on three different batches of nanoparticles to test reproducibility.
All fractions were relatively non-toxic when compared with literature values for cytotoxic nanoparticles. There didn’t appear to be any direct correlation between particle size and cytotoxicity, but certain fractions were reproducibly more toxic than others.
To investigate this effect in more detail, we tested two hypotheses: (1) specific particles may have specific effects within the cell and (2) specific particles may be taken up more efficiently by the cells.
We first managed to falsify hypothesis (1) by identifying the toxicity mechanism as involving reactive oxygen species in the cell, which was detected for both the more and less toxic nanomaterials, just to a lesser extent in the less toxic ones.
Hypothesis (2) was tested by measuring the degree of liposome encapsulation for size-separated particles. It turned out that the more toxic fractions were able to incorporate into the liposomes more efficiently than the less toxic fractions.
These results suggest that the observed differences in cytotoxicity are due to enhanced cellular uptake of certain lipophilic fractions.
This discovery could lead to purification of specific nanoparticles capable of enhancing therapeutic potency by increasing the amount of therapeutic material that enters target cells. The understanding gained by this work opens the possibility for screening nanoparticle libraries for biological activity in the same way that is currently done in drug discovery with chemical libraries, which is what the scientists plan to do next.
This is an essential step toward the discovery of novel nanotechnology based therapeutics.