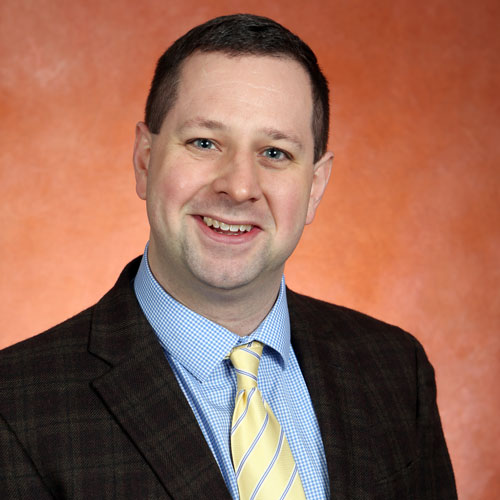
All cells, from bacteria to humans, must be able to rid themselves of damaged or unneeded proteins in order to remain healthy.
Understanding how cells perform this vital task has become a source for discovering new and potentially more effective ways of treating diseases ranging from cancer to neurodegenerative disorders.
The process is a research focal point for Robert J. Tomko Jr., assistant professor of biomedical sciences at the Florida State University College of Medicine. He recently received a $1.5 million National Institutes of Health grant to dig deeper into how cells dispose of their protein waste.
The endpoint for these proteins is the proteasome, a “machine” assembled within cells and responsible for removing unwanted debris. Many human diseases are caused or sustained by either too much or too little proteasome activity. Turning the proteasome off has shown great promise in the treatment of several cancers, whereas turning it back on could help treat many neurodegenerative diseases.
The proteasome consists of three functional units: a processing unit (or lid); a motor (or base) that uses cellular energy to power the machine; and a core particle, capable of breaking chemical bonds within proteins.
Once these three units assemble, they form a fully functional protein-destroying machine. The three units then work together to process proteins destined for destruction: The lid removes the delivery signal from the incoming proteins, the base unfolds them into amino acid strings and the core particle then chops those strings into little pieces.
“We want to understand how the individual contacts between these different units allow them to coordinate with one another, so that as they each do their respective jobs to process proteins that need to be destroyed, they can make sure they are in step with one another,” Tomko said.
He likens it to gears fitting together in an engine.
“For it to work, each step has to happen in the appropriate order and in a coordinated fashion,” he said. “We want to know how that happens. Right now, we have pictures of what the proteasome looks like during different steps of this protein processing, but we don’t really know how we get from State A to State B to State C.”
A key element in understanding the process is determining what causes it to fail. Inactive proteasomes and the resultant accumulation of toxic, damaged proteins are a hallmark of many neurodegenerative disorders, such as Alzheimer’s and Parkinson’s. Understanding how the proteasome is activated could provide important information on how it could be turned back on in patients suffering from these diseases.
“Our goal with this research really is to try to understand the events that drive proper activation of the proteasome,” Tomko said. “How is the signal transmitted throughout the entire machine from a spot that is, in many cases, highly localized and away from the parts that actually receive it? And then to see if we can devise clever ways to either trigger that activation or prevent it with small molecules or other approaches that could be further developed into therapeutics.”
The key, according to Tomko, is to understand what drives the proteasome’s relay system.
“If we know how it works, we can make it work to our advantage in fighting diseases,” he said.